The name “stem” cells came from plant stems, which, despite their tiny size, have the capacity to produce flowers, leaves, branches, fruit, vegetables, and gigantic trees. In the same way, stem cells, although microscopic, contain the potential to develop into different body parts — to repair or replace diseased or injured cells. Stem cells can differentiate, which means they can become a retina or pancreas cells, skin cells or shin cells, cells specific to the nose or to the toes. Stem cells are sustained by a microfluidic environment of supporting blood vessels and channels for other fluids. And a stem cell’s microfluidic environment influences the decision about what body part it will become.
New research suggests microfluidic factors might also determine whether the stem cell is stressed or not stressed. Stem cells that are growing and developing under stress from radiation treatment were observed to differ from stem cells developing in less-stressed environments— that is, with no radiation. A recent animal study suggests stem cells might be able to switch between a “normal-growth” version of themselves, to a “growth-under-stress” version, if that is what the body needs.
Dr. John Chute, of the UCLA Broad Stem Cell Research Center and a professor of hematology/oncology, investigates differences between the microfluidic environments of normal-growth stem cells, compared to those of growth-under-stress stem cells. The Chute team’s goal is to illuminate why normal-growth cells can switch to become growth-under-stress cells, as reported in a recent Cell Stem Cell article. In a press release, Chute observed that “Although the switch occurs, the reason for the change is a mystery.”
Treatments such as radiation and chemotherapy leave human blood-forming cells dangerously stressed and depleted. A growth-under-stress version of stem cell treatment might be able to heal that depletion much better than a normal-growth version.
The new findings spur questions about whether it might be possible to predict the stress level of stem cells, and to administer the most effective stem cell treatments to radiation patients, in order to speed recovery.
Just as soil nutrients sustain a plant stem, the microfluidic environment surrounding stem cells nurtures them. According to Chute, “In stem cell research, two important questions are, ‘What are the micro-environment cells that regulate stem cells?’ and ‘How do they do it?’” U.S. National Institutes of Health scientists agree: “Microfluidics offers a systematic way to study the decision-making process of stem cells.” In addition, analyses of stem cells based on the microfluidics that nurture them “can be done in a much deeper and wider way” than without them.
NIH scientists have also observed that it is ultimately their microfluidic complexities that predict how stem cells will become one particular body part or another. To gain a precise understanding of how body-part differentiation happens, microfluidic analyses are a necessity.
That necessity is nowhere more evident than in efforts to find out how switching between normal-growth and growth-under-stress stem cells happens. The possible impact on recovery from cancer treatment could be immense. That impact supports industry analyses that the microfluidics market, for which the 2016 global evaluation was $4.76 billion U.S. dollars, will grow to $12.45 billion by 2025.
Numbers tell the story. A surging biotechnology sector paired with the simultaneously increasing global burdens of disease are estimated to drive up market growth. For example, according to the 2017 World Health Organization data, the number of patients suffering from diabetes worldwide was estimated at 422 million in 2014 — and microfluidic advances contribute to innovative diabetes treatments.
The endless frontier of research targets also points to growth on many fronts, from stem-cell stress levels to brain cells, fibrosis and bone joints.
Currently, some new companies that develop stem cells for brain research — deliverable to scientists in both industry and academia — are gaining clients because many facilities do not have the resources to generate neural stem cells themselves. The new companies assemble stem cell types onto microfluidic chips that duplicate human tissue, as well as predict physiological processes. As their novel production challenges are ironed out, these companies will develop and deliver in short time frames.
As reported in a recent Financial Post article, Canada’s Genomics Application Partnership Program (GAPP) supports collaborations specifically to bridge the gap between research and commercialization, and is now funding a $6.5 million microfluidics project to develop fibrosis treatments.
According to U.S. National Institutes of Health scientists, the limitations of a bone joint and cartilage repair are fueling the development of stem cell therapies for weakened cartilage, and this work relies “upon microfluidic technology.”
Predictions about microfluidic commercialization encompass the fact that there were more than 15.5 million cancer survivors in the U.S. in 2016, and this number might be more than 20 million by 2026. About 7 million U.S. patients have had bone treatments such as hip or knee replacements. By 2030, U.S. cartilage-related knee replacement surgeries are projected to total 3.5 million per year. More than 70,000 people worldwide live with cystic fibrosis.
Analysts need only to do the math.
Enjoyed this article? Don’t forget to share.
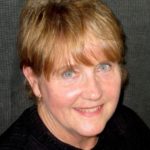
Kathy Jean Schultz
Kathy Jean Schultz is a freelance medical science writer who focuses on medical innovations. She earned a Master’s Degree in Research Methodology from Hofstra University, and a Master’s Degree in Psychology from Long Island University. She is a member of the National Association of Science Writers, and the Association of Health Care Journalists. Her articles about organoids include "Would you trust a 3-D printed mini organ to test your drugs?" and "Stem cells not only slow disease, they come with their own safety test".