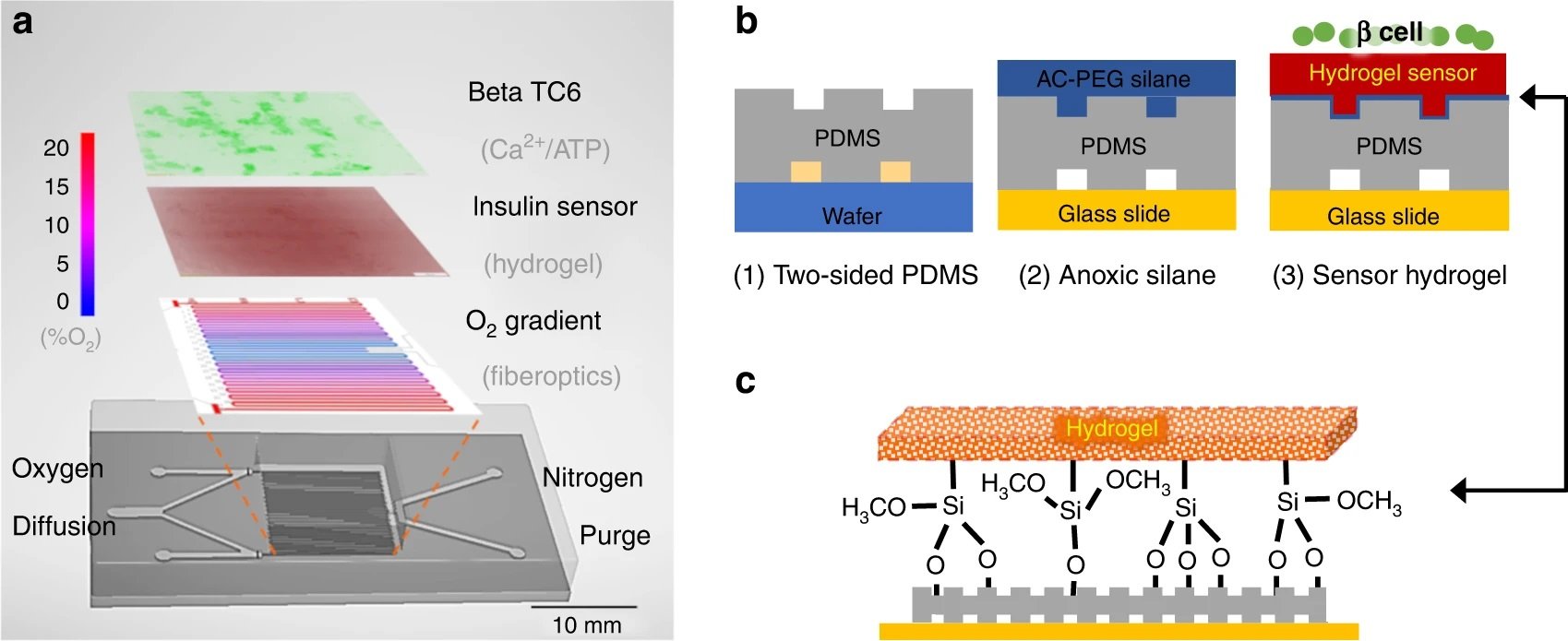
13 Feb Multilayered Microfluidic Chip for Spatiotemporal Detection of Pancreatic β-cells Secretions under Oxygen Gradients
Recent advances in microfluidic devices have brought about exciting new possibilities in the field of cell assays. From drug discovery to basic research, microfluidic chips are paving the way for more efficient and effective studies in the field of cell biology. These devices allow for greater control and manipulation of cells, and can be used to create complex gradients or concentrations for a wide range of applications. In this week’s research highlight, we delve into a recent paper published in Microsystems and Nanoengineering that explores new ways in which microfluidic devices are being utilized in cell assays, and the benefits they offer over more traditional methods. This paper presents a novel approach for studying pancreatic beta cells using a multilayered microfluidic device with an integrated oxygen gradient and a hydrogel sensor. The microfluidic device was microfabricated to address the limitations of conventional microfluidics in combating diffusion, which limits the spatial distance and time for molecules traveling through microchannels. The microfluidic platform leverages a rotational fluid shear apparatus to assess gel adhesion on polydimethylsiloxane (PDMS) and enables gradient-based multimodal biosensing, which allows the modulation of both oxygen and aqueous factors on beta cell cultures.
“Here, we leveraged a multilayered microfluidic approach to integrate a novel oxygen gradient (0–20%) with an enhanced hydrogel sensor to study pancreatic beta cells. This enabled our microfluidics to achieve spatiotemporal detection that is difficult to achieve with traditional microfluidics. “, the authors explained.
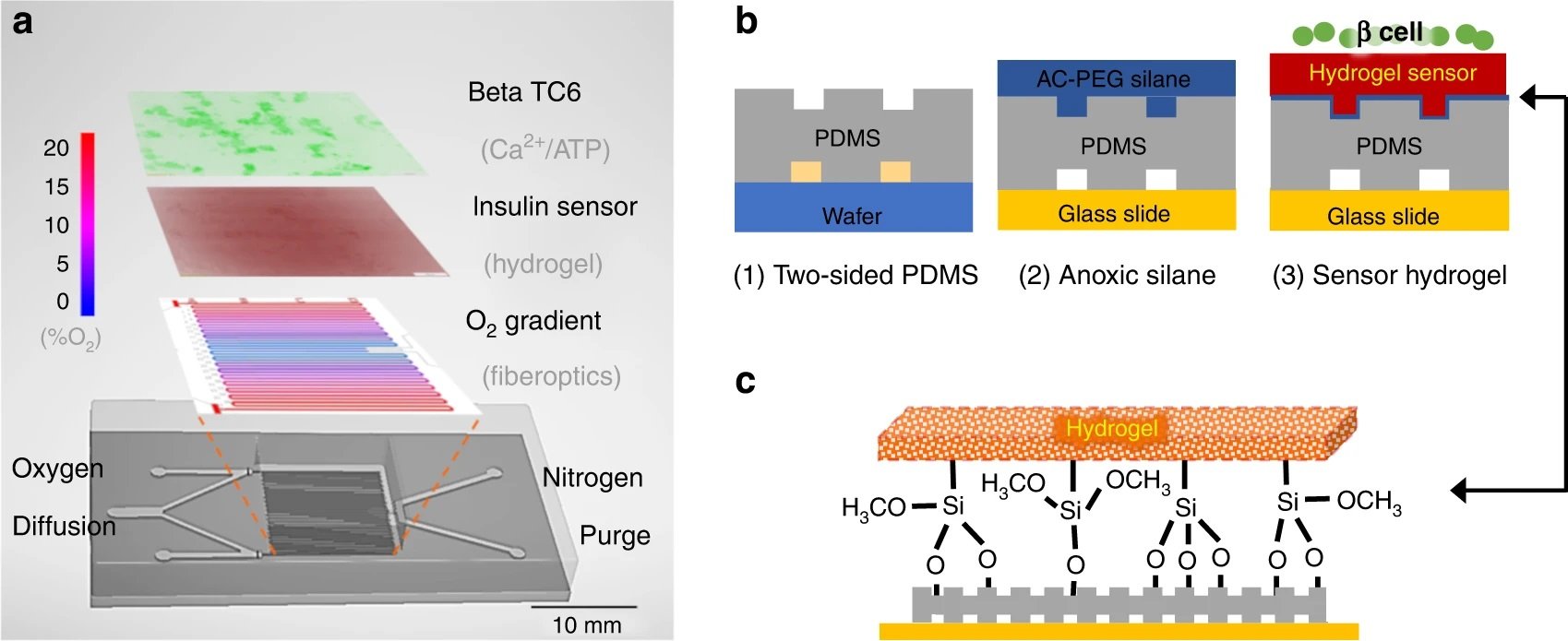
“a The microfluidic device contains multiple layers. The gas layer balances oxygen diffusion (red) with nitrogen convection (blue) to create a 0–20% gradient as measured with a fiber optic oxygen probe. Note that the gradient is symmetrical, so either half can be used. The 200 µM polydimethylsiloxane (PDMS) membrane that defines the gas channels is highly permeable to oxygen and diffuses the gradient to the hydrogel and cell culture seeded atop. On top of the oxygen gradient, a porous hydrogel sensor was bonded to the microchannels. This hydrogel encapsulates anti-insulin capture antibodies, 20k polyethylene glycol (PEG) porogen, and arginylglycylaspartic acid (RGD) peptides to provide cell attachment and insulin detection. The open top aqueous reservoir allows normal cell culture processes and fluorescence microscopy. b The fabrication process of the multilayered microfluidics begins with normal photolithography and PDMS molding. During the chemical surface modifications, a nitrogen chamber is used to prevent oxygen inhibition of hydrogel cross-linking at the PDMS–gel interface. The resultant sandwich is washed with buffer to remove uncross-linked reagents and porogens to complete the porosity-enhanced hydrogel sensor. c Moreover, attachment of the functionalized hydrogel is achieved through both topological and chemical surface modifications. Microtextures are patterned on the cell facing the side of the PDMS membrane to increase the surface area in contact. Then, the sandwich is incubated with acrylated silane to create Si–O-bonds to tether the hydrogel backbones” Reproduced under Creative Commons Attribution 4.0 International License from Duan, K., Zhou, M., Wang, Y. et al. Visualizing hypoxic modulation of beta cell secretions via a sensor augmented oxygen gradient. Microsyst Nanoeng 9, 14 (2023).
The device’s spatiotemporal detection capability allows for in situ stimulation and detection, which eliminates variability between individual bioassays. The microfluidic platform allows for the detection of calcium, insulin, and adenosine triphosphate (ATP) in response to glucose and oxygen stimulation. The hydrogel sensor’s high porosity and direct path from the cell to the sensor improve insulin detection, with levels as low as 25 pg/mL quantified using an imaging technique. The device also enables the monitoring of calcium and mitochondrial ATP levels in situ, revealing interesting dynamics at approximately 10% oxygen levels.
The platform’s application can be extended to numerous oxygen-dependent tissue dysfunctions, and it can be adapted for discrete protein detection in a robust platform. The device provides a robust technique for gradient-based multimodal biosensing and can be applied to numerous shear-dependent cellular studies. The spatiotemporal detection capability of the device is achieved using a multilayered microfluidic approach, which integrates an oxygen gradient and an enhanced hydrogel sensor to study pancreatic beta cells.
The paper also provides insights into the specific effects of oxygen levels on glucose-stimulated insulin response (GSIR) and islet therapies. The observed optimal oxygen level of 12% for calcium oscillation goes against the conventional view of a linear relationship between hypoxia and GSIR. The study also suggests new strategies for improving islet therapies, including the use of microfluidics for islet selection and preconditioning to hypoxia. Furthermore, gradient modulation of calcium oscillation can train naïve β-cells or stem cell-derived islets to synchronize their GSIR for a more responsive replacement tissue.
“The microfluidic technique enables experimental protocols to further answer these questions and provides a platform to apply training strategies for islet therapies. Furthermore, the technique for 3D fluid shear stress can provide a facile hydrogel adhesion test for biomaterial applications. “, the authors explained.
Figures and the abstract are reproduced from Duan, K., Zhou, M., Wang, Y. et al. Visualizing hypoxic modulation of beta cell secretions via a sensor augmented oxygen gradient. Microsyst Nanoeng 9, 14 (2023). https://doi.org/10.1038/s41378-022-00482-z under Creative Commons Attribution 4.0 International License
Read the original article: Visualizing hypoxic modulation of beta cell secretions via a sensor augmented oxygen gradient